To say that hysteria about the health effects of polyunsaturated fats [PUFA] has reached fever pitch may be an understatement.
It can be interesting watch a trend of thought gain traction when that train of thought is inconsistent with a substantial total body of evidence, from multiple converging lines of inquiry.
It speaks to something at play beyond scientific discourse, and frankly much of the “debate” regarding PUFA is steeped in a mix of narratives, from fantasies about our evolutionary past to conspiracy theories about governments, scientists, the food industry, and ‘BigPharma’.
I have a few thoughts as to why the PUFA-hysteria appears to have exponentially increased in recent years. It’s likely not an exhaustive list, but these are some of the more common narratives I’ve observed.
1) The surge in popularity of “ancestral” viewpoints of health, arguments based on assumptions about our evolutionary diet, and the popularity of modern versions of the ‘Paleo’ diet.
The populist, modern incarnation of the ‘Paleo’ diet seems divorced from the evidence. Pay attention to any ‘guru’ in this area, and you’ll inevitably hear an emphasis on saturated animal fats, as if this nutrient formed a backbone of the ancestral diet. Except, this romantic image of our caveman forebears sitting around the slain wooly mammoth gorging on red meat washed down with a Bulletproof Coffee is not what the evidence suggests. Far from it: it appears the idea that our evolutionary diet was rich in animal fat is an evolutionary fantasy dreamed up by modern wannabe-cavemen, unsupported by any real evidence. Saturated fat are estimated to have provided about 6% of the average total energy intake for humans in the Paleolithic period, reflecting the fat composition of wild game meat in free-living African mammals. And over half – up to 60% – of the brain’s dry weight is lipid (fat), and of that up to 30% is comprised of polyunsaturated essential fatty acids. Docosahexaenoic acid [DHA] alone comprises over 90% of omega-3 fats acid in the brain, generating a “shore-based perspective” of human evolution.
Stable isotope analysis of bones of early Sapiens have indicated that marine protein sources constituted significant proportions of daily energy in the human diet. Anthropological evidence for the expansion of the genus Homo from the African rift valley suggests a migration along coastal and inland watercourses, provided access to both marine and freshwater sources of fish, and in particular long-chain omega-3 fatty acids. The evidence suggests that the consistent access to such food sources coincided with the period of exponential encephalisation, preceding the rapid development of language, complex reasoning, and problem solving cognitive capacities associated with the prefrontal cortex. Relative to other mammalian species, and indeed our primate cousins, the human brain is disproportionately large compared to body size. Other mammals with high levels of other polyunsaturated fats in membranes, but without a direct dietary source of preformed DHA, did not develop large brains, indicating a foundational need for preformed DHA in the early modern human diet evident in the preference for DHA and polyunsaturation in neural membranes.
2) Ability to find rodent studies to corroborate beliefs about PUFA.
Sure. It is completely uncontroversial that in many rodent studies, lipid peroxidation has been demonstrated relative to the degree of unsaturation of fats in the experimental feed, and the antioxidant capacity of the host animal. It’s odd that such studies in our rodent friends are used to support theoretical arguments of human evolution harking back to the Palaeolithic period. To say nothing of their lack of translational evidence in humans. Human outcomes > rodent mechanisms. That is how we evaluate strength of evidence.
3) Play on ‘BigFood-BigPharma-wants-to-make-you-sick’ emotive narratives.
I’m not going to defend the food industry and the role it has played in altering the food environment and deliberately propagating both bad science and a poor food supply. But that argument can still be made grounded in facts and evidence, not emotively-driven conspiratorial beliefs. The idea that PUFA have been injected into our food supply to contribute to a slow and painful demise is nonsense rhetoric. This also tends to come hand-in-hand with the “it all started with Ancel Keys” diatribe, regurgitated by automatons who haven’t even read his research, or even of the wider diet-heart literature, from that period. I’ve written an extensive article on the early metabolic ward studies from that period, and the various research groups involved, which you can read elsewhere on site.
4) It’s easy to build a pseudoscientific case against PUFA.
This is most assisted by citing rodent studies without attempting to build a cohesive case or argument. These arguments may seem impressive to the unsuspecting reader. They’re often referenced, which provides a veneer of evidence-based. But closer scrutiny and it’s just a collection of standalone claims with a rodent reference from 50yrs ago at the end. “PUFA have been shown to do X (Rat et al., 1972)”. “Y increases when PUFA are fed (Mice et al., 1965)”. No attempt to cogently synthesise the arguments and look for converging lines of evidence. Then state potential mechanisms as if they’re established in vivo effects, followed by some dazzling with chemistry language as if translational research doesn’t exist. And not to mention, flat out ignore an entire of body evidence of outcomes in humans. Sprinkle on top the usual conspiratorial icing, and it all sounds rather impressive, but the argument is a house of cards.
In addition to the 50yr old rats, we’ve had a resurgence of some dusty old human studies which add to the pseudoscientific veneer: Sydney Diet-Heart and the Minnesota Coronary Experiment in particular like to do the rounds. Citing studies like these involves either total cognitive dissonance or lack of scientific literacy, both of which are mainstream issues within the ‘carnivore’ community and other low-carb iterations. Recently, I’ve also seen reference to the Women’s Health Initiative and the Helsinki Businessman’s Study: we’ll address these briefly when we discuss these studies further, below. Oh, and of course you can also do a meta-analysis with some of these studies, and just like the bad data that goes in, bad data comes out.
The Claims
The gravamen of the argument for PUFA being potentially deleterious for human health centres on two processes: inflammation and oxidation, specifically, lipid peroxidation. In a nutshell, the argument centres on the chemical composition of PUFA resulting in these fatty acids with double-bonds being prone to lipid peroxidation, and therefore adverse effects through oxidative stress. Additionally, that the omega-6 linoleic acid acts as a precursor to pro-inflammatory eicosanoids, and therefore that PUFA cause adverse effects through inflammation.
We’ll look at human evidence in relation to both, but first, let’s discuss a concept that is critical to understand when assessing claims about diet and health: putative mechanism does not equal certain effect.
However, this assumption between putative mechanism – or basic chemistry – and assumed effect underpins much of the anti-PUFA rhetoric. This assumption relies on a rhetorical tactic where a statement of fact is offered as evidence of a conclusion. Let’s take an example of when Cholesterol Denialists argue “but the body NEEDS cholesterol, bro!” and talk about ‘building blocks’, as if this fact provides evidence of a conclusion that we need high levels of cholesterol. But the science-based reality is that very little amounts are physiologically normal, and we really don’t need that much.
The exact same rhetorical hack is deployed with much of the claims regarding PUFA. “Well you see they’ve a double-carbon bond at the omega-6 position” as if this provides evidence of a conclusion of disease risk in humans, or “well you see linoleic acid is a precursor to arachidonic acid, and that is pro-inflammatory”, as if this provides evidence of an actual inflammatory effect. What they are not telling you, or asking, is the answer to the fundamental question: is this physiologically relevant in humans? Does this putative mechanism translate to an in vivo effect?
Again, from the perspective of the lay person these types of arguments can sound scientific and convincing. But let’s deal with the fundamental question: what is the evidence for these processes in humans as a result of PUFA intake, and are these results relevant?
Inflammation and Lipid Peroxidation (In Humans)
Let’s start with inflammation. The putative mechanism with regard to inflammation is that the omega-6 linoleic acid [LA] acts as a precursor to arachidonic acid [AA]; AA acts a substrate to form eicosanoids, and the eicosanoids AA is used to form may be pro-inflammatory. Ergo, as the logic goes, increasing PUFA – particularly LA – increases inflammation.
Except there is no evidence that either increasing or decreasing LA levels alters levels of AA in humans. A review of 36 human intervention studies highlighted that neither increasing LA levels by up to 551%, or decreasing LA levels by 90%, altered concentrations of AA in plasma, serum, or red blood cells [erythrocytes], despite increasing LA intake resulting in increased membrane phospholipid LA content. Putative mechanism does not = biological effect. The following illustration helps to illustrate why:
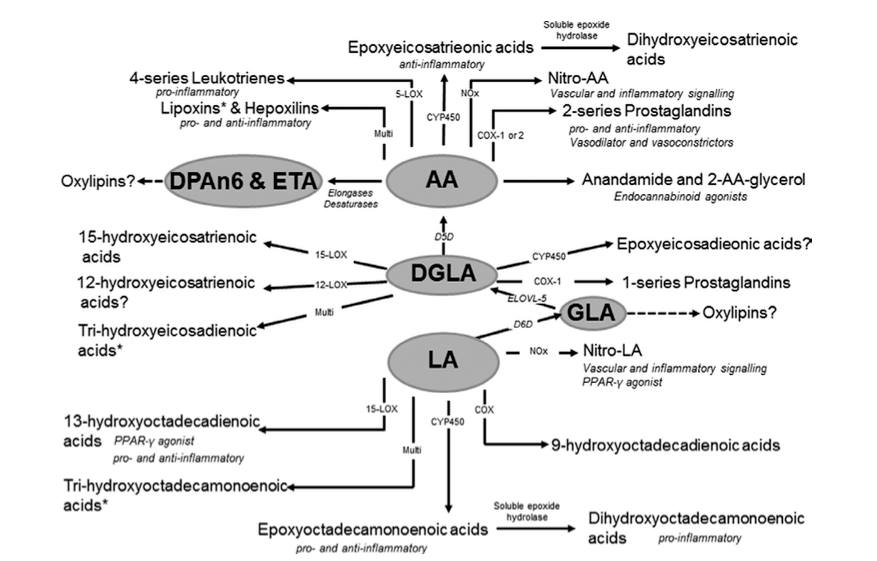
The delta-6-desaturase [D6D] enzyme responsible for converting dietary essential fatty acids into longer-chain fatty acids, is also necessary for conversion of LA to AA, and in adulthood there is very little activity of D6D. As you can see from the above figure, LA is also not directly metabolised to AA, but goes through multiple intermediate conversion processes, which require D6D. The net effect is that AA levels are kept at a relatively constant level in the body, and this is also observed during breastfeeding, where milk is maintained with a constant AA level through triglyceride lipolysis, despite dietary intake.
That is the first step in the putative mechanism of inflammatory effects of LA – conversion to AA – for which there is no evidence of dramatic changes in LA dietary intake altering circulating levels of AA. What about inflammation itself? Johnson & Fritsche conducted a review of 15 human intervention trials investigating the effects of LA on inflammatory biomarkers. Their conclusion was that “virtually no data are available from randomised, controlled intervention studies among healthy, non-infant human beings to show that the addition of LA to diets increases markers of inflammation.”
This is where the buck just stops for the inflammation argument. There is no evidence that the putative mechanism is operative, nor that there is an inflammatory effect of dietary LA in actual Homo Sapiens. And, let’s not forgot both the anti-inflammatory effect of alpha-linolenic acid [ALA] and the anti-inflammatory role of EPA and DHA.
So, what of lipid peroxidation? A search of the terms “polyunsaturated” and “lipid peroxidation” and “human” in PubMed reveals a small number of trials that have directly tested the effects of PUFA on various markers or surrogate markers of lipid peroxidation in our very own species.
The studies vary greatly in terms of methodology, intervention, and most importantly, the assessment of lipid peroxidation, so a meta-analysis would be pointless. Instead, I’ve qualitatively synthesised the studies. To reiterate: these are studies in actual humans.
Strapans et al., 1994: 6 healthy males were tested in an acute, single meal postprandial study. The control diet was corn oil that was unoxidised plus high vitamin E, compared to two diets with moderate and high levels, respectively, of oxidised lipids together with low vitamin E. These diets contained ~57-59% LA. The results demonstrated that levels of oxidised lipids from the pre-oxidised oils determined levels of oxidised lipids in postprandial chylomicrons. High LA unoxidised corn oil with high vitamin E content (0.14mg per gram oil) had no effect on any of the measured markers of lipid peroxidation. While this study indicates that oxidised oils may be absorbed from dietary intake, the low vitamin E content of the oxidised lipid diets likely biased the results, as vitamin E is an important modifier of lipid peroxidation. More recent evidence suggests consumption of oxidised fish oils does not increase more reliable markers of oxidative stress. Further, the use of indirect assays to measure lipid peroxidation, including thiobarbituric acid reactive substances (TBARS) and conjugated dienes, which are non-specific and may measure products other than lipid peroxidation, and be elevated without concomitant elevations in markers of oxidative stress.
Turpeinen et al., 1995: This study conducted two separated studies, using both in vivo and in vitro analyses. Both studies compared a sunflower oil diet to a rapeseed oil diet. In study 1, 59 healthy adults underwent strict dietary control with a sunflower oil diet containing 13% PUFA [12% LA] compared to a rapeseed oil diet containing 7% PUFA, 5% LA, and 2% alpha-linolenic acid [ALA], against a high saturated fat milk-fat based diet. The milk-based diet was followed for 14-days, followed by 24-days on the sunflower oil diet then rapeseed oil diet for a further 24-days. Half of the participants completed the study in reverse diet order. There were no significant differences in malondialdehyde (MDA) levels, an end-product of lipid peroxidation, or indirect lipid peroxidation assays, including TBARS or conjugated dienes. In the second study, 13 healthy adult participants were assigned to the sunflower oil or rapeseed oil diet to follow free-living for 6-weeks. In this study, levels of TBARS and lipid peroxidation measured in lipoproteins tended to decrease in the sunflower oil diet, although this was only statistically significant for LDL, and exhibit no change in the rapeseed oil diet. However, the in vitro analysis revealed the opposite effect: the PUFA-rich sunflower oil resulted in increased oxidation. This is an important finding because it demonstrates that oxidation may be demonstrated in vitro, which does not correspond with measured in vivo effects. This could have been attributable to the high vitamin E content of the diets, particularly the sunflower oil PUFA diet, with the implication that antioxidant availability in vivo is a more relevant determinant of potential lipid peroxidation.
Jenkinson et al., 1999: This was a free-living study in 10 healthy males comparing diets with 5% PUFA to 15% PUFA, with carbohydrates, fats, protein, and vitamin E matched. Again, this study used outcome markers that are not reliable measures of oxidative stress, in particular TBARS. The authors themselves highlight the non-specific nature of this assay, and the fact that it may measure byproducts that do not result from lipid peroxidation. The study did find that oxidised glutathione was higher on the 15% PUFA diet, however, there was no difference in total or reduced glutathione, or red blood cell glutathione. The finding in relation to oxidised glutathione may reflect an insensitive measure used in this study. Concentrations of oxidised glutathione are very low, and changes may not necessarily reflect oxidative stress. Accurately measuring oxidised glutathione requires high-performance liquid chromatography, which was not conducted.
Södergren et al., 2001: This was a free-living study in 19 healthy adults with diets provided to participants, comparing a rapeseed oil enriched diet to a saturated fat enriched diet. Each diet was consumed for 4-weeks, separated by a 4-week washout period, with participants randomised to order of intervention. The study used reliable measures of in vivo oxidative stress, specifically isoprostanes, in addition to malondialdehyde (MDA). There were no significant difference in markers of oxidative stress or lipid peroxidation, while circulating levels of g-tocopherol increased significantly during the rapeseed oil diet, suggesting the levels of antioxidant naturally present in the oil is sufficient for protective effects.
Nielsen et al., 2002: This was a controlled study in 18 healthy males measuring in vitro effects of three diets enriched with rapeseed oil, olive oil, or sunflower oil, respectively. Compared to the olive and rapeseed oil diets, the sunflower oil diet increased susceptibility of VLDL and LDL to oxidation. However, this was in vitro, and this effect of sunflower oil in vitro was also observed in the study above by Turpeinen et al, who found the opposite, i.e., no effect in vivo.
de Kok et al., 2003: This was a supplemental intervention in 30 healthy females comparing two levels of LA intake, 15g/d and 7.5g/d, to 15g/d palmitic acid, taken for 6-weeks. MDA and markers of oxidative DNA damage were the primary outcomes, and there was no increase in any marker of oxidative stress or DNA damage at any level of LA intake.
Egert et al., 2007: This was a controlled dietary intervention in 75 young adults comparing 3-weeks on rapeseed oil diets enriched with ALA, EPA, and DHA, respectively, after controlled 2-week matched diet run-ins. The study oils contained 7.5% omega-6 and 2.5% omega-3 PUFA, and the oils contained high vitamin E content. Ex vivo oxidation of LDL was the primary outcome. ALA did not effect LDL susceptibility to oxidation, while the effects of EPA and DHA were inconsistent. LDL susceptibility to oxidation was greatest during the DHA-enriched diet. To reiterate a theme, this study is yet another example of the need for caution in extrapolating results from in vitro or ex vivo models to an in vivo effect, and the need to ask whether the finding could be physiologically relevant in vivo. DHA and EPA may have had, albeit inconsistently, effects on LDL susceptibility to oxidation ex vivo in this study: reconcile that against the plethora of data from prospective cohort studies, tissue biomarker studies, and intervention studies, demonstrating the benefit of these PUFA for cardiovascular and neurodegenerative disease.
Freese et al., 2007: This was a feeding study in 77 healthy adults with diets controlled for 6-weeks, comparing diets of 11% and 3% LA for effects on lipid peroxidation and oxidative DNA damage. Isoprostanes measured oxidative stress, and the study measured an in vivo nucleotide which reflects oxidative DNA damage. MDA, TBARS, and ex vivo measures of LDL susceptibility to oxidation were also analysed. Overall, there were no significant differences in any marker of oxidative stress, lipid peroxidation, or oxidative DNA damage.
The foregoing studies are interventions which have directly tested the effects of PUFA, either in diet or supplements, on markers of lipid peroxidation and/or oxidative stress. It is important to be sensitive to method, as many studies have used indirect or non-specific assays, which may not in fact measure lipid peroxidation or reflect oxidative stress. More recent studies using isoprostanes, which have strong utility as biomarkers of oxidative stress, have not demonstrated oxidative effects of PUFA in vivo. Arguably the most important overall finding to synthesise from those studies is the difference between in vitro or ex vivo models, and actual in vivo effects. The disconnect reflects the difficulty with extrapolating results from in vitro studies to assumed effects in vivo, and collectively it appears that there is little evidence from controlled intervention studies that lipid peroxidation from PUFA intake, of various total PUFA intake and varying LA levels, is a major cause for concern in vivo. This is a crucial point in the interpretation and representation of the evidence regarding PUFA.
With the inflammation and oxidation claims addressed, let’s turn to the oft-cited studies proffered as evidence from interventions in humans of an adverse effect of PUFA.
The Oft-Cited Human Studies
Most people would likely never have heard of the Sydney Diet Heart Study or the Minnesota Coronary Experiment if not for a recent re-analysis of both studies, and a meta-analysis based on these studies and other studies which used linoleic acid [LA] interventions, like the 1965 Rose et al. Corn Oil Trial. These flawed analyses are now celebrated as conclusive evidence within low-carb/ancestral/paleo/carnivore circles for apparently uncovering “tHe tRUth”. Except there is one major problem from an evidence-based perspective: reanalysing old, flawed studies with methodological shortcomings and missing data doesn’t make them better studies. You can’t put lipstick on a pig, and you certainly can’t take a pig, donkey, and giraffe and attempt to put lipstick on them together in a meta-analysis.
Let’s start with Sydney Diet-Heart Study [SDHS]. The SDHS was a randomised controlled trial conducted between 1966 to 1973 in 458 men aged 30-59 years who had suffered a recent coronary event (myocardial infarction, acute coronary insufficiency, or angina). Baseline SFA intake was ~16% and PUFA ~6%; the intervention group were advised to increase PUFA intake to 15% energy by replacing SFA [lowering SFA to <10%]. The intervention was provided to participants in the form of a safflower oil and safflower margarine. Median follow-up time was 3.3yrs, the original study only reported on all-cause mortality, for which there was a statistically insignificant increase in risk [HR 1.62, 95% CI 1.00-2.6]). The Ramsden et al. reanalysis also published results for cardiovascular disease, indicating statistically significant increased risk for CVD mortality [HR 1.70, 95% CI 1.03-2.80] and coronary heart disease mortality [HR 1.74, 95% CI 1.04-2.9]. The confidence intervals are, however, so wide as to indicate weak statistical power and render difficulty inferring where a true effect may lie.
But let’s focus on the major problems with the SDHS. First, two distinct intervention foods were provided: an oil and a margarine. We have no data on whether participants consumed more of one or the other, but the increased mortality in the intervention group lends a clue. Hydrogenated fats added to oils to make commercial margarines at the time contained up to 25-40% of fatty acids as trans-fats. The margarine used in the study, Marrickville Margarine from the brand ‘Miracle’, was a safflower oil-based margarine produced for commercial use. And the intervention group substantially increased PUFA intake with the provided foods, likely consuming substantial amounts of trans-fats – which are unequivocally associated with significant increased risk for CVD. Moreover, attributing this effect to LA is not possible, because while the oil may have had a high LA content, margarines at the time were predominantly monounsaturated fats, together with the high trans-fat content. There are so many unknown variables with this study in terms of the exact fatty acid composition of the diet, other than the broad label of ‘PUFA’, which is likely an inaccurate description of diet in the first place given the aforementioned factors. And the bottom line is that no one is in a position to dispel the potential confounding, because we will never have that data. In that regard, SDHS simply shouldn’t be cited when better quality more recent data exists.
So how do we reconcile it? Against the wider evidence-base, of course. We can start with a meta-analysis from 2014 which conducted a subgroup analysis of 8 omega-6 LA intervention trials, finding no significant effect including the SHDS: removing the SDHS in a sensitivity analysis resulted in a 21% reduction in CVD risk from LA intake [HR 0.81, 95% CI 0.68-0.98]. But we’ll save the rest of the evidence base for the next section, first let’s put a fork in the Minnesota Coronary Experiment [MCE].
Not satisfied with dredging up the SDHS, the authors went looking for more data to dust off and reanalyse. The reanalysis, published in the BMJ (obviously, anything that runs with heart disease revisionism is a lock for publication), was of another intervention conducted in the mid 1960’s in 9,057 patients attending state psychiatric hospitals in Minnesota. The control diet was a diet high in saturated fat, compared to the intervention diet designed to be high in PUFA, and study meals were provided to patients while they were in the institution. The outcomes were blood cholesterol levels and coronary heart disease events. In the original study, there was a statistically insignificant 8% [HR 1.08, 95% CI 0.84-1.37] relative risk increase in risk for coronary events in the intervention group. The confidence intervals in this study are spread across the null so as to make the results practically meaningless to interpretation. The Ramsden et al. re-analysis looked at the associations between blood cholesterol levels and risk of CHD mortality, in 2,355 participants with baseline and follow-up cholesterol measurements. This reanalysis suggested that a decrease in cholesterol of 0.78mmol/L [30mg/dL] was associated with a statistically significant 22% [HR 1.22, 95% CI 1.14-1.32] relative risk increase of all-cause mortality, only in participants aged over 65yrs, irrespective of which diet they were assigned to.
Here is the thing: MCE was a poorly executed study by any standards. Participants were not exclusively in-patients; many were in and out of hospitals, meaning that their participation in the intervention was not continuous, and they consumed study meals while in hospital, before returning to their day-to-day lives. For these participants who were in and out of hospital, the cholesterol measurements were taken after 3-weeks back on the diet following reentry. Without knowing what their levels were upon reentry to the hospital, it is possible that the period after reentry influenced the results. Let’s be really clear about what the MCE, and the reanalysis of the cholesterol levels, can support: nothing. It is incorrect to state that the MCE is evidence of an increase in risk from PUFA, because the original trial findings were insignificant and spread across the null. It is incorrect to state the the PUFA intervention resulted in lower cholesterol and increased mortality risk, because the same effect was observed in the high SFA group. And, finally, the fact that, irrespective of diet, the risk of increased mortality risk with lower cholesterol levels was only in the >65yo age group participants implies what Rose and Shipley termed the “unsuspected sickness phenomenon”, characterised by an underlying disease condition resulting in lowering cholesterol levels as the metabolic consequence of the disease. This is a common occurrence in this age group, an observation that allows some to play statistical gymnastics trying to show that higher cholesterol is associated with “longevity”.
Time out for a quote:
“No statistical technique can compensate for fundamental limitations of the input data.”
This quote by Professor Sander Greenland is referring to meta-analysis, and this is apt for the next study because, as if the reanalysis of SDHS and MCE wasn’t enough, Ramsden et al. conducted a meta-analysis with these two trials and one more, the Corn Oil trial from 1965. And to reaffirm the quote above, a meta-analysis does not solve any fundamental problems in the input data; if the input data is bad, the output is worse. As stated, the relative risk and confidence intervals in MCE render the interpretation of the study meaningless, while the Corn Oil trial resulted in a relative risk of 4.64 [95% CI 0.62-37.15]. The overall meta-analysis of these trials was insignificant, and the near-significant association was based largely on the direction of effect in the SHDS reanalysis, and Rose Corn Oil trial. One has to beg the question, with the preponderance of data available from multiple, converging lines of evidence, what purpose a meta-analysis of three studies from the mid-1960’s with the limitations identified above served.
Forest plot from Ramsden et al. meta-analysis illustrating barn-door confidence intervals, low statistical power, and effects of incorporating flawed input data on GIGO [garbage input, garbage output].
The final two studies to be briefly mentioned, which I’ve recently seen added to the SDHS and MCE as proof that replacing SFA with PUFA apparently increases CVD risk, are the Women’s Health Initiative and the Helsinki Businessman’s Study. In the WHI, participants in the intervention group were counselled to reduce saturated fat to ~8% energy, and increase wholegrains, fruit and vegetable, and PUFA intake. While the intervention group managed to reduce SFA from 12.7% to 9.5% over 6yrs, nothing else was achieved for the intervention diet. PUFA decreased from 7.8% to 6.1%, and carbohydrates from refined sources increased. The lack of effect of lowering SFA is explainable by the replacement with largely refined carbohydrate, as evident in the wider literature. So the WHI is categorically not evidence that increasing PUFA has any adverse outcome. Finally, the Helsinki Businessman Study. The 5-year multifactorial intervention in men with elevated cardio-metabolic risk factors advised participants to replace SFA, cholesterol, sugar, with PUFA (mainly as soft margarine), fish, chicken, and vegetables, in addition to physical activity, medications, and smoking cessation. Despite significant reductions in risk factors, incidence of coronary events tended to be higher in the intervention group than in the control group – 19 vs. 9 cases, respectively. However, the analysis of the study indicated that the increase in mortality risk had nothing to do with diet. Univariate and multivariate analyses indicated that the increased risk in the intervention group was associated with use of beta-blockers and clofibrate. Incidence of CHD was lower in the groups who used diet only.
Ultimately, we are talking about a handful of human intervention studies, mostly from 40-60yrs ago, which purport to find a particularly detrimental effect of PUFA, and LA in particular. They are methodologically flawed, mostly statistically insignificant, infer no confidence in the statistical power of the findings, and have critical variables – like trans-fats – which can never fully be accounted for. But even if we assumed for a minute that they were sound studies, they would still only be a handful of studies in the context of a wider literature accumulated from multiple lines of inquiry, from metabolic wards to prospective cohorts to RCTs, which all converge to support a benefit to PUFA. That is how conclusions are derived in science; not from a rat, a single study, or a handful of studies, but an accumulated total body of evidence.
Converging Lines of Evidence (In Humans)
Ecological data. Prospective cohort studies. Tissue biomarker studies. Metabolic ward studies. Intervention trials. Meta-analyses. In humans.
Metabolic Studies:
This body of evidence dates back to the tightly controlled metabolic ward studies in the 1950’s conducted by various research groups at the time. This period is also important, because within the scientific community there was already evidence that industrial hydrogenation might alter the physically properties of a fat or oil, in turn changing its physiological effects. Here is an example of this from Bronte-Stewart et al., published in The Lancet in 1956, which indicated the difference in blood cholesterol levels in response to groundnut oil vs. hydrogenated groundnut oil:
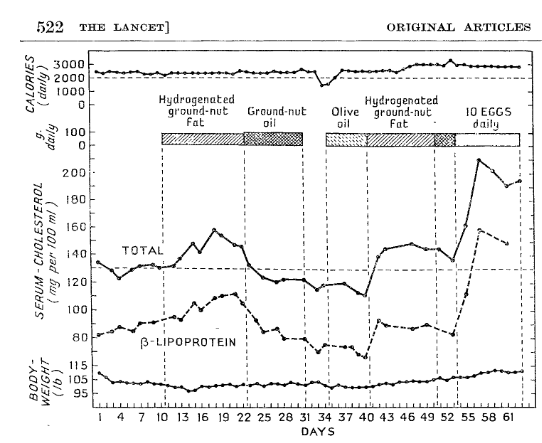
This effect of hydrogenation was evident in a number of studies from these various research groups at the time, highlighted in the following quote from a review from Page et al. published in JAMA in 1957:
“The parameter of saturation of fat as a significant factor in cholesterolaemia and, by extension, in atherosclerosis, recently has been explored in human beings by Kinsell, Ahrens, Beveridge, and Bronte-Stewart. These experiments showed that relatively unsaturated fats of vegetable or marine origin tend to lower while, per contra, the particular hydrogenated vegetable fats usually, and saturated animal fat rather regularly, tended to increase serum cholesterol.” [Emphasis added].
Thus, the effects of hydrogenation of vegetable oils was evident early in this literature. As we know, however, unfortunately this did not translate into food industry practices for decades.
Keys et al. spent six years experimenting with controlled diets in metabolic studies, varying only in the type and quantity of dietary fat [from 9-44% energy], which ultimately resulted in the ‘Keys Equation’, a predictive equation for the impacts of saturated, monounsaturated, and polyunsaturated fats, and dietary cholesterol, on blood cholesterol levels. The Keys Equation remains valid today. The most important contribution from the Keys Equation was identification of the ‘P:S ratio’, i.e, the ratio of polyunsaturated to saturated fats in the diet, as the most important factor determining blood cholesterol levels in response to diet. To quote from Keys et al. in The Lancet in 1957:
“The experiments reported here clearly indicate that the saturated fatty acids, at least those of chain length longer than 10 carbons, have about twice as much effects in raising the serum-cholesterol level as the cholesterol depressing effect of an equal amount of polythenoids [polyunsaturated] or linoleic acid.”
Good science is reproducible, so let’s jump forward by several decades to two meta-analyses of nearly 400 metabolic ward studies, Hegsted et al., 1993, and Clarke et al., 1997. Hegsted et al. confirmed the validity of the Keys Equation in a regression analysis modelling the effects of dietary fatty acids and cholesterol on blood cholesterol levels, in a synthesis of metabolic ward studies published up until 1991. To quote:
“There is little reason to believe that maximal effects of polyunsaturated fatty acids on serum cholesterol are reached at modest polyunsaturated fat intakes.”
Clarke et al. published a meta-analysis of 390 metabolic ward studies modelling both univariate effects [i.e., each dietary constituent in isolation], and multivariate effects [i.e., the effects of substitution/relationship between dietary constituents], demonstrating unequivocally that the effects of isocaloric replacement of energy from SFA with PUFA has the greatest impact on reducing LDL-cholesterol, triglycerides, and overall blood lipid profiles.
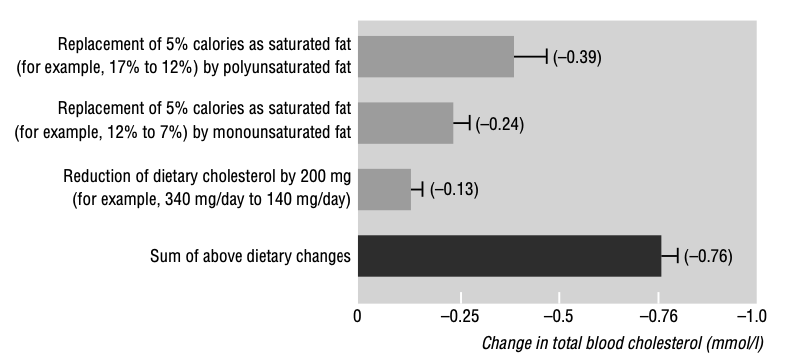
Figure from Clarke et al. demonstrating the effects of isocaloric replacement of saturated fat with unsaturated fats, and reduction in dietary cholesterol, on blood cholesterol. A clear hierarchy is evident with polyunsaturated fats exerting the greatest lipid-lowering effect.
As short-term metabolic ward studies conducted over periods of 2-8 weeks, these studies are looking specifically at intermediate risk factors. In this regard, they provide mechanistic plausibility to the effects on cardiovascular disease reduction from the replacement of SFA with PUFA evident in long-term prospective studies.
Ecological Studies:
It doesn’t necessarily take controlled feeding studies to observe the effects of replacement in the diet. There have been some interesting ecological observations occurring as a result of political upheaval, and also population-wide public health initiatives. Let’s start with the observations from Eastern Europe following the fall of the Soviet Union, in which certain countries experienced a rapid decline in CHD/CVD rates, attributed to a period of market transformation and expanding of the food supply. This sharp decline in heart disease mortality was correlated with a significant increase in population intake of the PUFA, alpha-linolenic acid [ALA]. Traditionally in Eastern Europe, rapeseed or sunflower oil were the predominant oils, but rapeseed oil – rich in both monounsaturated omega-9 and polyunsaturated omega-3 – had been more expensive under Communist regimes, and thus animal fats or sunflower oil, which is low in ALA, remained the predominant cooking fats and oils. Market transformation made rapeseed oil more accessible, and its consumption increased significantly in Poland, Latvia, Lithuania, Czech Republic, and Slovakia: these countries with the greatest increase in grams per day of ALA showed the greatest reduction in mortality. In contrast, Russia, Bulgaria and Romania, which showed no increase in ALA, had no change in CVD mortality.
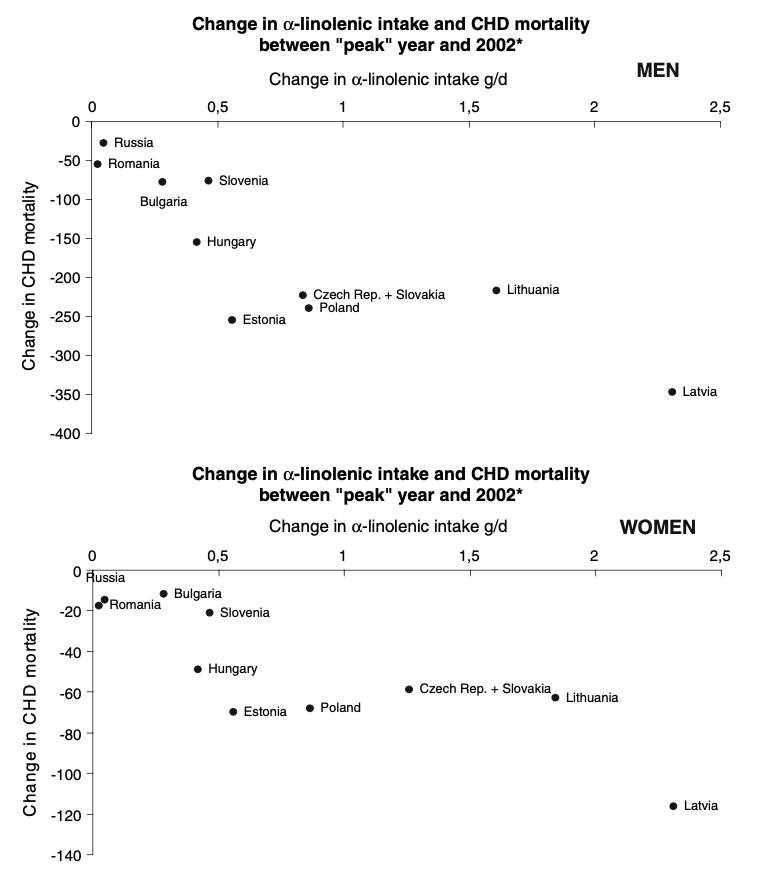
Figure from Zatonski et al. depicting changes in ALA intake and associated changes in CHD mortality in former Soviet countries after 1991.
While the observation in Eastern Europe resulted from external and unforeseen political forces, starting in the early 1970’s Finland embarked on a targeted public health campaign to reduce CHD/CVD mortality. In the 1960’s Finland had the highest population SFA intake in the world at 21-23% energy, with the highest population blood cholesterol levels, and experienced the highest CHD/CVD mortality globally. Starting in 1972, a public health intervention to reduce mortality targeted population reductions in specific risk factors, in particular smoking rates, blood cholesterol, blood pressure, and adiposity. By 2007, CHD/CVD mortality had declined by 80% and of the cumulative risk factors, population reductions in blood cholesterol accounted for 67% of the decrease in mortality. A primary determinant in the population-wide reduction in blood cholesterol was a reduction in dietary saturated fat from 21-23% to 12-13%, achieved through deliberate public health messaging regarding butter consumption [the most significant contributor to SFA in Finnish diets at the time], with dietary advice to replace SFA with PUFA [amongst other dietary advice, including increased vegetable intake and lean meats over fatty meats]. Of particular note, this decrease occurred in the context of smoking rates remaining largely similar [slight decline in men, increase in women], and an overall increase in BMI across the population, both significant risk factors for CVD.
These are ecological studies and population-wide observations, so the usual caveats apply. The Finnish example is also not wholly attributable to PUFA, given that SFA have twice the cholesterol-raising effect as PUFA have lowering cholesterol, i.e., the reduction in SFA across the population was the strongest determinant of reducing population-wide cholesterol levels, and heart disease mortality. Nonetheless, these ecological observations are congruent with the wider literature, both prospective and interventional, and thus have value in the synthesis of converging lines of evidence.
Prospective Cohorts:
In a 23-year mortality follow-up of the Israeli Ischemic Heart Disease Study in 10,059 male civil servants published in 1993, using LA to determine the P:S ratio indicated that the greater the P:S ratio, i.e., the greater the ratio of LA to SFA, was associated with reduced CHD mortality, as was LA alone as a percentage of total fat intake [confidence intervals were not shown]. Further, adjustment for blood cholesterol levels rendered null the findings in relation to dietary fat, indicating that the relationship between the P:S ratio and CHD mortality was mediated by blood cholesterol levels, as would be predicted from metabolic ward studies.
In 2009, Jakobsen et al. published an analysis of data pooled* from 11 cohorts, including 344,696 participants with a range of from 1.7% to 10.6% PUFA intake 9.4-21.3% SFA intake, over 4-10yrs of follow-up in which 5,249 coronary events and 2,155 coronary deaths occurred. Replacing 5% of energy from SFA with PUFA was associated with a 13% relative risk reduction for coronary events [HR 0.87, 95% CI 0.77-0.97] reduced risk for coronary deaths by 26% [HR 0.74, 95% CI 0.61-0.89].
*In a pooled analysis, the individual data from the participants in the included studies is combined – ‘pooled’ – together. This provides increased statistical power, and allows for testing different aspects of the relationship between an exposure and outcome.
In a meta-analysis by Farvid et al. including 12 cohorts in the US, Europe, and Israel, comparing high vs. low intakes [median 6.4% to 1.5%, respectively] of LA was associated with a 15% [HR 0.85, 95% CI 0.78-0.92] relative reduction in risk for CHD events, and 21% [HR 0.79, 95% CI 0.71–0.89] reduction in risk for CHD death. In dose-response analysis the relationship between increasing LA as a percentage of energy and lower risk of CHD events and deaths was linear: each 5% increase in LA was associated with a 10% [RR 0.90, 95% CI 0.85–0.94] lower risk for events and 13% [RR 0.87, 95% CI 0.81–0.93] lower risk for CHD mortality.
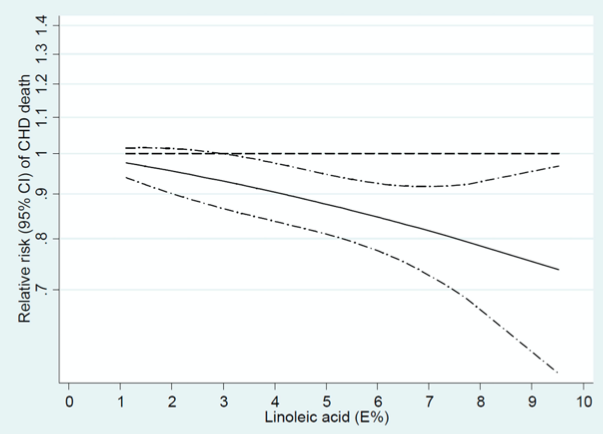
Figure from Farvid et al. illustrating linear association between increasing linoleic acid (as a percentage of energy) and reduction in risk for coronary heart disease.
This linear association was observed again by a paper published this year by Li et al., a meta-analysis including 21 prospective cohorts, 811,069 participants, 50,786 CVD deaths, and follow-up median duration from 4.9yrs to 30yrs. PUFA intake ranged from 1.1% to 11.6%, and each 5% increment of energy intake from LA was associated with a 7% [RR 0.93, 95% CI 0.91-0.95] reduction in CVD risk.
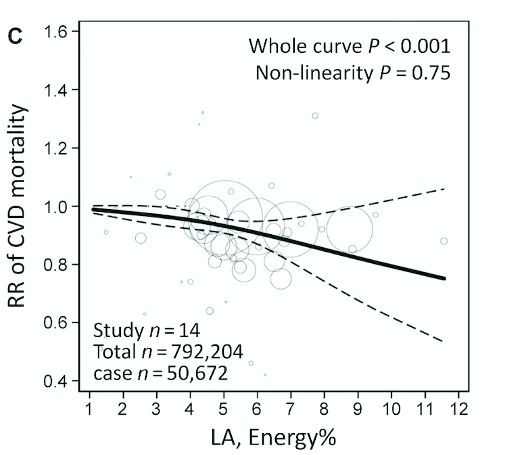
Figure from Li et al. illustrating linear association between increasing linoleic acid (as a percentage of energy) and reduction in cardiovascular disease mortality risk.
Finally, in a combined analysis of the Nurses’ Health Study and the Health Professionals Follow-Up Study, substitution of 5% energy from SFA with PUFA generally was associated with a 25% reduction in CHD mortality. Of particular note in this analysis was the hierarchy of effects of substation: if you contrast this with the figure from the Clarke et al. meta-analysis of 395 metabolic ward studies above, you’ll see that the effect of substitution mirrors that of the magnitude of effect of substitution on blood lipids. While there is no doubt more going on in the picture of cardio-metabolic health, this relationships provides biological plausibility to the reductions in CVD and CHD risk observed by increasing PUFA intake, particularly where it displaces dietary SFA.
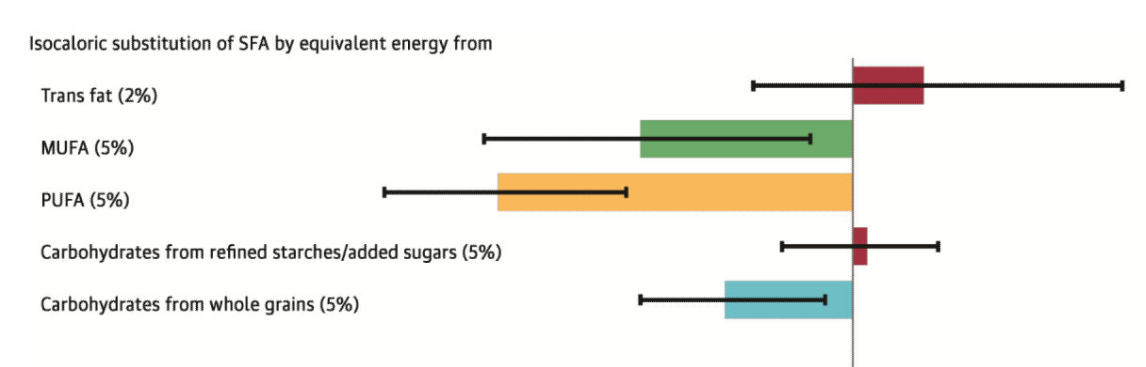
Figure from combined analysis of the NHS and HPFS by Li et al., illustrating the reduction in risk for coronary heart disease mortality associated with isocaloric replacement of saturated fat with other fat subtypes and carbohydrate subtypes. Replacement hierarchy evident as PUFA>MUFA>Complex Carbohydrate, consistent with data from metabolic ward studies of effects of replacement on blood lipids.
And what about dementia and Alzheimer’s Disease? In the Dutch Doetinchem Cohort Study, EPA and DHA were together associated with a 19% [OR 0.81, 95% CI 0.66-1.00] reduction in risk. These associations were not observed for other polyunsaturated fats, including the precursor to EPA and DHA, ALA, which suggests a particular role for EPA/DHA in the prevention of cognitive decline. This finding was repeated in the Chicago Health and Ageing Project [CHAP] study, which found that while neither ALA or EPA were associated with reduced risk, DHA specifically was associated with significant reductions in Alzheimer’s Disease risk [RR 0.30, 95% CI 0.10-0.90].
Cumulatively, the direction of effect in long-term prospective studies is consistent across populations in reducing risk for CVD and CHD outcomes, both events and deaths. This relationship has been shown to be linear for LA in separate analyses. In addition, the epidemiology on oily fish consumption and the long-chain omega-3 fatty acids, EPA and DHA, is one of the strongest associations for protective effects of diet against dementia and Alzheimer’s Disease. Thus, from this body of prospective data we can state that the relationship is temporal, strong, consistently significant in the direction of reduced risk, and exhibits a dose-response. All of these factors support a benefit derived from dietary PUFA. Now let’s proceed to tissue biomarker studies.
Tissue Biomarker Studies:
Biomarker* studies are primarily prospective cohort studies, but are highly valuable pieces of the evidential puzzle.
The term “biomarker” means use of a specific biochemical measure that provides an indication of nutrient intakes. This isn’t always as straightforward as “nutrient in = nutrient measured”, because nutritional status is influenced by variations in the digestion, absorption, metabolism, distribution, and excretion of a nutrient, which differs from nutrients to nutrient. For example, when measuring fatty acids, whether it is the phospholipid content of cell membranes, lipoproteins, or adipose tissue measured, each will provide different indications of dietary intake. Biomarkers may be classified according to what measurement they allow for.The most commonly used biomarkers, which measuring the concentration of a nutrient in a plasma, red blood cells, adipose tissue, are known as “concentration biomarkers”, as they are measuring the concentration of that particular nutrient in the circulation or tissue. How well a fatty acid correlates to dietary intake is highly dependent on the particular fatty acid that is analysed. This is because certain fatty acids cannot be produced in the body, while others can. So, if we look at the essentially fatty acid omega-3’s – ALA, EPA, & DHA – or omega-6 LA, phospholipid content of these fatty acids will better reflect dietary intake. Certain saturated fatty acids with even chain lengths (for example 14, 16, or 18-carbon length) may be synthesised in the body, however, and do not correlate well to dietary intake. The correlation also depends on the circulating factor analysed, because different measures reflect different time points of dietary intake: blood lipids reflect more recent intake within a number of days, while phospholipids may reflect a time-period of months, and adipose tissue up to 1-2yrs. The use of biomarkers is very attractive for nutritional epidemiology, as it allows for a reflection of dietary intake for certain nutrient markers that is independent of measurement error in the dietary assessment .
In the Fatty Acid and Outcome Research Consortium [FORCE] of studies comprising 30 cohorts in which 76,356 fatty acid measurements of circulating or adipose tissue fatty acids from 68,659 participants across 13 countries, pooled analysis demonstrated that the highest levels of tissue LA were associated with a 23% [HR 0.77, 95% CI 0.69-0.86] reduction in risk for total CVD. Plasma levels of the dreaded arachidonic acid were also associated with a significant 21% [HR 0.79, 95% CI 0.67-0.93] reduction in risk for total CVD. But this had been previously demonstrated, in a meta-analysis of 10 studies on biomarkers of AA levels, circulating levels were associated with a 17% relative reduction in CVD risk [RR 0.83, 95% CI 0.74–0.92].
In the US Cardiovascular Health Study, in participants followed-up over 34,291 person-years of follow-up in which 1,994 deaths occurred, higher plasma phospholipid LA levels were associated with a 13% [HR 0.87, 95% CI 0.74–1.02] lower risk of all-cause mortality, albeit not statistically significant. In a Finnish cohort study, higher serum levels of LA were associated with a significant 46% [HR 0.54, 95% CI 0.40-0.74] relative risk reduction comparing the highest to lowest circulating biomarker levels. In the recent meta-analysis by Li et al., data on biomarkers of LA and mortality outcomes was available from 28 prospective cohorts, including 65,411 participants. In the analysis of all tissue compartments combined, increased tissue LA concentrations in pooled analysis was associated with a 11% [HR0.89, 95% CI 0.85-0.94] reduction in risk for CVD.
Forest plot from Li et al. demonstrating overall effect size associated with linoleic acid measures in various tissue compartments, and overall effect size.
Similarly, biomarker studies have provided mechanistic corroboration of prospective cohort findings in relation to dementia/AD. In the Framingham Offspring Study, participants with the lowest red blood cell DHA levels displayed significantly lower total cerebral brain volume and performed worse on cognitive testing, compared to the highest DHA levels. In the Women’s Health Initiative Brain MRI Study, women had MRI scans conducted 8yrs after baseline RBC omega-3 samples were collected. High baseline Omega-3 Index [combined DHA+EPA] levels correlated with a 2.1cm larger brain volume determined by MRI. This is, however, not to suggest that there is no role for EPA in protection against cognitive decline. EPA may be low in brain tissue itself, however, higher plasma levels of EPA have been associated with a 31% [HR 0.69, 95% CI 0.48-0.98] reduced depression-associated dementia risk, and higher cerebral plasma EPA levels associated with prevention of brain grey matter atrophy in the amygdala.
By conducting analyses of biomarkers of PUFA intake, i.e, objective in vivo measures of fatty acids, these studies add a level of certainty from epidemiology, where an RCT with thousands of participants and objective measures of diet would be infeasible. Nevertheless, there are people who will dismiss all of the foregoing in favour of rats and Sydney-Diet Heart, so let’s wrap this up with evidence from the hallowed sanctity that is the randomised controlled intervention trial.
Intervention Studies:
This is where the data comes full circle and appears unequivocal. In 2010, Mozaffarian et al. conducted a meta-analysis of controlled feeding studies with a median of 4.25yrs duration. In the overall pooled analysis of eight RCTs, there was a 19% [RR 0.81, 95% CI 0.70-0.95] relative reduction in risk for CHD events. Subgroup analysis by trial duration indicated that in interventions >4.25yrs there was a 27% [RR 0.73, 95% CI 0.61–0.87] reduction in CHD event risk. In substitution analysis, a mean increase of 10% PUFA in the intervention groups indicated that each 5% energy from SFA replaced with PUFA reduced LDL-C by an average of 0.25mmol/L [10mg/dL], and CVD risk decreased by 10% [RR 0.90, 95% CI 0.83–0.97]. The mean cholesterol reduction in the intervention arm compared to the control arms was was 0.76mmol/L [29 mg/dl], corresponding to a 24% [RR 0.76, 95% CI 0.62–0.93] reduction in CHD event risk for each 1mmol/L [38.6mg/dL] reduction in total cholesterol levels. Of particular note in this comprehensive meta-analysis was the correlation between the relative risk reduction for CHD from predicted changes in blood cholesterol from intervention studies, the reduction in CHD events in intervention studies, and the reduction in CHD risk observed in data from the Jakobsen et al. pooled analysis cited above.
Figure from Mozaffarian et al. illustrating relative risk of coronary heart disease events from PUFA replacing SFA, including predicted effects on blood cholesterol from short-term interventions, the meta-analysis of interventions, and the data extracted from the pooled analysis by Jakobsen et al.
And we can look at the Hooper et al. updated meta-analysis of intervention studies from this year, which found that the replacement of SFA with PUFA was associated with a 27% [RR 0.73, 95% CI 0.58-0.92] reduction in risk for CVD events. We could look at the REDUCE-IT [Reduction of Cardiovascular Events with Icosapent Ethyl–Intervention Trial], which found that a high-dose EPA supplement given to patients in secondary prevention [i.e., they had already suffered a cardiovascular event] led to a 25% reduction in risk for a further cardiovascular event. We could look at the recent Hu et al. meta-analysis of omega-3 supplemental interventions in secondary prevention, which included REDUCE-IT, and found an 8% [HR 0.92, 95% CI 0.86-0.98] reduction in risk for CHD death and 12%[HR 0.88, 95% CI 0.83-0.94] reduction in risk for myocardial infarction. In dose-response meta-analysis, each 1g/d EPA/DHA resulted in a 17% [HR 0.92, 95% CI 0.88-0.97] reduced risk of CVD death.
The practical implication is that, in my opinion, anyone attempting to support an argument that replacing SFA with PUFA increases heart disease risk either hasn’t done their due diligence or is being wilfully ignorant of the totality of evidence because studies like Sydney Diet-Heart confirm their preconceived bias.
Conclusions
In order to sustain the case that PUFA intake is deleterious to human health, anyone advancing the argument has to provide a rebuttal to the multiple lines of evidence from RCTs, prospective cohort studies, and biomarker studies, which all converge to the same conclusion. They can’t.
In order to sustain such a case, the putative mechanisms of lipid peroxidation and inflammation have to be shown to relate to adverse health outcomes with supporting evidence of sufficient quality in humans. It isn’t there.
And in order to sustain such a case, the handful of studies that are cited in support of such an adverse effect in humans need to stand up to scrutiny of their methodology. They don’t.
A total body of evidence: ecological studies, prospective cohort studies, tissue biomarker studies, metabolic ward studies, intervention studies, and meta-analyse respectively of cohort studies, biomarker studies, metabolic ward studies, and intervention studies. Every line of evidence converges to a conclusion in favour of the role of polyunsaturated fats, including the omega-6 linoleic acid, and health outcomes in humans.